
When rotor downwash hits the ground surface, the vortex circulation is outward, upward, around and away from the main rotors in all directions.
Helicopter wake turbulence is more complex than wake turbulence caused by a comparably sized airplane due to its different wake structure, duration and decay. These characteristics create a potent threat, especially around airports where helicopters are engaged in low speed flight while nearby fixed-wing aircraft are landing or departing.
An airplane in the takeoff or landing phase is at a slow airspeed which lessens the power of the flight controls to counter an abrupt motion, and the airplane has essentially no altitude margin for recovery from an upset caused by a wake encounter.
These were the conditions that existed at Cable Airport (CCB) in Upland, California, on Jan. 3, 2022. The pilot of a Cessna 120 was on approach to land while a UH-1 “Huey” helicopter was conducting a slow hover taxi adjacent to the runway. The Cessna 120 pilot decided to land long to maintain separation, but when the helicopter appeared to cross the runway, he decided to go-around.
About one-third down the runway, the Cessna 120 encountered the helicopter’s downwash and entered an uncommanded steep right bank. The pilot attempted to counter this roll with opposite aileron, but it was insufficient to countermand the induced roll. A video of the actual sequence of events can be found on the Flight Safety Foundation’s Aviation Safety Network website.
The Cessna 120 impacted right of the runway and sustained substantial damage. The pilot fortunately sustained only minor injuries. The NTSB determined the pilot’s loss of control during the go-around was due to a wake turbulence encounter from a slow hover taxiing helicopter.
Airplane Rolls Close To Ground
A similar event occurred on Dec. 5, 2014, at Northern Colorado Regional Airport (FNL), outside Fort Collins, Colorado, for a solo student pilot of a Cirrus SR20. The student pilot entered the traffic pattern for a full-stop landing on Runway 33. He observed a Sikorsky UH-60 Black Hawk on downwind and delayed his turn to base leg until the helicopter was on final, abeam his position. The student pilot adjusted his aim point to land long due to his concern about wake turbulence. His goal was to land beyond the helicopter’s touchdown point.
During the initiation of the landing flare, the SR20 suddenly rolled into a steep left bank. The student reacted by attempting to go around but the nearly instantaneous roll close to the ground resulted in an impact with the terrain. The airbag seatbelt assemblies mounted in the two front crew seats did not deploy as the airplane cartwheeled. The student pilot was seriously injured and the aircraft was substantially damaged.
A YouTube video captured this segment of both the departure of the Black Hawk and the abrupt roll of the Cirrus. It is readily apparent that the roll of the Cirrus happened so fast and so close to the ground that there wasn’t time now altitude for a recovery.
The NTSB investigation of the accident determined that the helicopter transitioned into a departure about 30 sec. ahead of the Cirrus, leaving behind an invisible wake of powerful vortices. The winds were relatively light at the time, recorded as 3 kts from 110 deg. In other words, this was a quartering tailwind.
The safety board’s report found that the student pilot likely did not comprehend the significance of wake turbulence created by a helicopter during the departure, which resulted in the loss of control during landing. The NTSB’s causal finding also determined the pilot guidance in the Aeronautical Information Manual and an advisory circular on aircraft wake turbulence published at the time did not recommend separation criteria for a small airplane following a helicopter.
After similar accidents the NTSB has noted that accident pilots likely did not comprehend the significance of wake turbulence created by a helicopter.
A Helicopter’s Wake Is Different
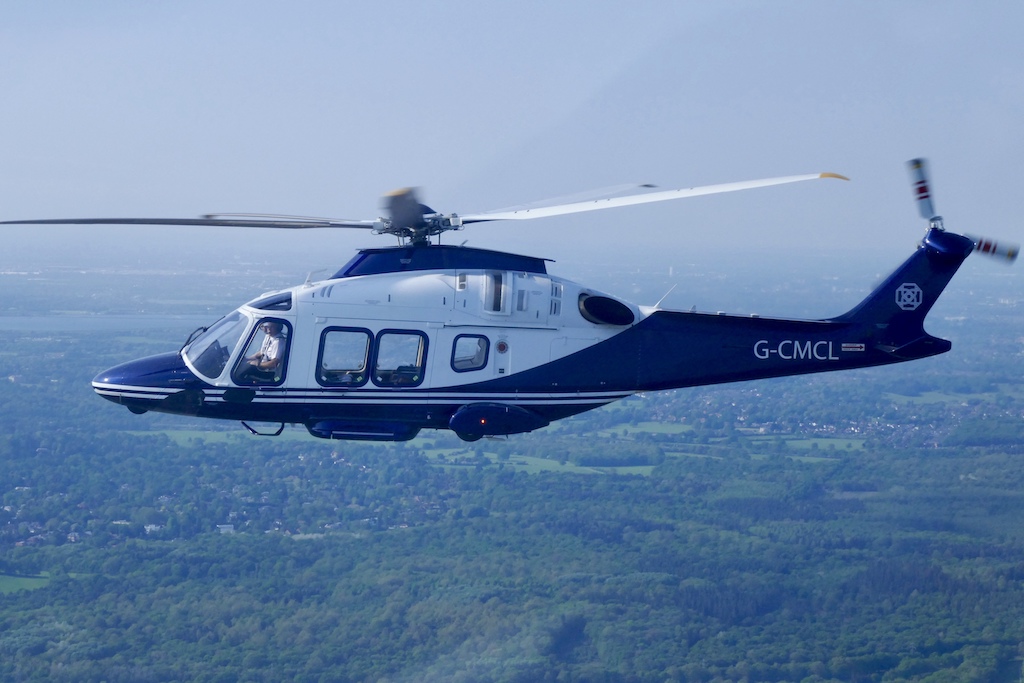
There are some similarities in wake vortices formed by fixed-wing and rotary-wing aircraft. Just like fixed wing aircraft, helicopter vortices are dependent on a helicopter’s weight, size and speed. Vortices formed at low airspeeds are initially stronger than those formed at higher airspeeds. A heavier helicopter produces stronger wake vortices than a lighter helicopter. The strength of a vortex is also dependent on its age.
Since each rotor blade is generating its own vortex continuously throughout a rotation, a helicopter’s wake is composed of a series of vortices that not only contain their own mini-tornado rotation but also the overall downward motion from the downwash. When rotor downwash from a helicopter in hover or slow flight close to the ground hits the surface, this turbulent airmass circulates outward, upward, around and away from the main rotors in all directions. A slow-moving helicopter’s wake is the equivalent of a small microburst, and must be given a wide berth.
The area contaminated by the wake turbulence of a helicopter is larger than that of an airplane of comparable size and weight, especially at speeds below 70-80 kts. A distance of approximately three times the diameter of the rotor contains substantial disruption from the downwash. The FAA’s Advisory Circular 90-23G “Aircraft Wake Turbulence,” advises pilots to avoid operations within distances of 3 times the diameter of a helicopter in a slow hover taxi or stationary hover.
The blade number appears to also effect vortex size, as an increased number of rotor blades appears to increase the vortex size. For example, the Bell UH-1H, with two rotor blades and essentially the same weight as the Sikorsky S-76A with four rotor blades produces a smaller vortex than the S-76A.
A helicopter’s wake in forward flight is more complex than the wake created by an airplane because each blade’s vortices will differ as its angle of attack varies throughout a single rotation depending on whether the blade is advancing or retreating in comparison to the approaching airflow. The retreating blade operates at a higher angle of attack in order to produce as much lift as the advancing blade. The vortex behind the retreating blade is characterized by a greater cross sectional area. The vortex behind the advancing rotor blade is consistently smaller, tighter and more coherent, especially as the helicopter’s forward speed increases above 80 kts.
Flight testing has discovered that helicopter wakes react differently depending on whether the helicopter is climbing or descending. The vortex cores were observed moving further apart during descents, while the cores would move closer together during climbing flight. A possible explanation includes the amount of engine power required to generate lift, and therefore the hot exhaust is entrained in the wake, therefore contributing to the buoyancy of the wake.
In Part 2 of this article, we describe FAA flight testing to better understand the risk to a fixed-wing aircraft that inadvertently flies into a helicopter’s wake.
Comments